Research
A detailed analysis of the evolution of languages from a neurobiological perspective, ranging from the genetic changes that have increased human brain size to a comparison with primates and changes shaped by cultural and social domains.
Research on the evolution of language has given rise to several interesting yet unanswered questions. Can language be explained by the same adaptive forces that shaped other cognitive abilities? Did language emerge as a product of increased brain complexity, or was language itself a driving force for brain evolution? Is the origin of language rooted in step-wise genetic change, or should we consider its evolution as a continuum? From a neurobiological perspective, the mystery of language evolution can be explored in diverse domains starting from molecular and cellular, all the way up to the social and cultural domains.
Language and the brain
It is widely accepted that some of the preconditions for language evolution must be traced back to the field of neurobiology. According to Cedric Boeckx, the “language-ready brain” emerged from developmental changes in brain morphology and neural circuitry that occurred in humans following the split from a common ancestor with Neanderthals and Denisovans (Boeckx, 2014; Boeckx, 2017; Boeckx and Benitez-Burraco, 2014). However, the biological competence for language was acquired as an evolutionary continuum. Indeed, some of its capacities are shared with other animals. Linguistic processes can be mapped onto the Faculty of Language in the Broad Sense (FLB), comprising sensory-motor and conceptual-intentional processes shared with other animals, and the Faculty of Language in the Narrow Sense (FLN), which refers to the uniquely human linguistic computation system (Hauser et al., 2002). For example, birds acquired the ability to sing in a manner that shows fascinating analogies to speech. Songbirds develop their species-specific songs during a critical period of devel- opment when interacting with their conspecifics is crucial. After this developmental period, songbirds produce defective songs, reminiscent of the difficulty in learning new languages during human adulthood. Language thus depends on deeply conserved neural and developmental substrates which include a variety of genes, cell types, and chemicals (Hauser et al., 2002). There are, however, substantial differences in the scale and mode of language acquisition between humans and primates. Namely, the rate and scale at which children build their lexicon are exponentially larger compared to non-human primates (Bloom, 1998; Hauser et al., 2002), opening the possibility for an independently evolved mechanism.
“Language thus depends on deeply conserved neural and developmental substrates which include a variety of genes, cell types, and chemicals.”
Hauser et al., 2002)
What neurobiological changes can have promoted such an explosion in the diversity and complexity of language in humans? To address this question, we will first focus on the genetic, cellular, and anatomical changes that occurred during human evolution, thus spanning vast biological scales ranging from molecules to whole organs. Finally, we will compare language abilities between modern humans and apes, addressing the questions of language evolution at the levels of an organism and an entire society.
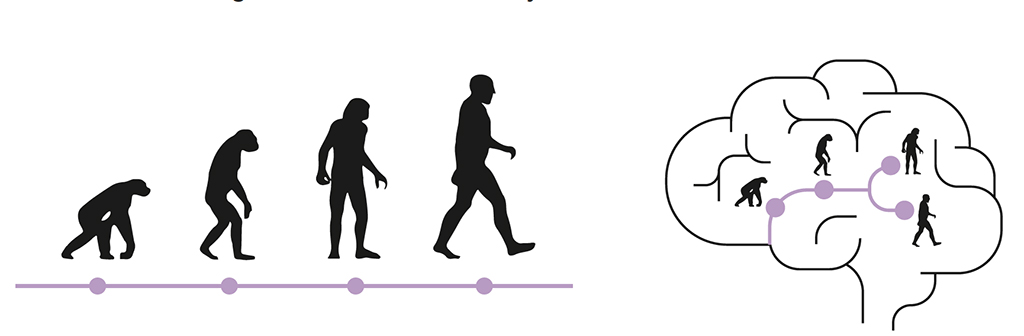
Genes
Our genes carry the blueprint of our body and are inherited across generations. Slight changes in genes, known as mutations, are responsible for intra- and inter-species diversity and are the main molecular drivers of evolution. Gene expression generates proteins, the main building blocks of our organs. Whereas all the cells of our body contain almost identical genomes, only certain tissues and specific genes will be expressed, and specific proteins will be made. Interestingly, our brain has more organ-specific proteins than any other part of the human body (Jiang et al., 2020).
If we compare the gene sequences of humans with those of other species, we can identify human-specific genetic changes or entire genes that developed recently in our evolution. On the other hand, if we compare the expression of human genes in the brain with other tissues, we can identify genes that play a role in brain function. Combining such information tells us which genes played a role in the evolution of the human brain. Such efforts resulted in the identification of ARHGAP11B and SRGAP2, truly human-specific gene duplications that evolved after the split from the chimpanzee lineage. ARHGAP11B is thought to be a key gene responsible for the increase in brain size in the human lineage (Florio et al., 2015). Indeed, adding this gene to mice, ferrets, and marmosets has resulted in an increase in their brain sizes, and in the case of mice, increased memory flexibility (Heide et al., 2020; Kalebic et al., 2018; Xing et al., 2021). On the other hand, SRGAP2 encodes a protein that promotes neuronal migration and the maturation of dendritic spines, which are both required for the proper connectivity of neurons in the brain (Charrier et al., 2012; Dennis et al., 2012; Guerrier et al., 2009; Sudmant et al., 2010). Although both ARHGAP11B and SRGAP2 may have a fundamental role in the steps that render the human brain a substrate prone to language acquisition, they are not directly involved in language evolution. The gene that shows the most direct link to language evolution is FOXP2, which controls the expression of other genes and hence has more widespread effects. Mutations in FOXP2 have been found in patients with developmental language disorders (Lai et al., 2001; MacDermot et al., 2005). Interestingly, there are two genetic mutations that set human FOXP2 apart from the chimpanzee version (Enard et al., 2002), and when these mutations are introduced into mice, they present altered vocalization and behavior (Enard et al., 2009; Schreiweis et al., 2014). It is likely that FOXP2 regulates this mechanism by controlling the expression of other genes which, in turn, control the production and connectivity of neurons (Tsui et al., 2013; Vernes et al., 2011).
From cells to organs
To understand how the activity of these and other genes translates to cellular and anatomical scales, we must further explore human brain development. Brain morphogenesis differs markedly in the period directly after birth in chimpanzees and humans (Gunz et al., 2010; Neubauer et al., 2010; Scott et al., 2014). Within the first year of life, only human skulls change rapidly from an elongated to a more globular shape (Gunz et al., 2010). The importance of this critical developmental window also lies in the development of synapses that help form a brain network by connecting individual neurons. During a critical window of postnatal development, external stimuli are fundamental in establishing a solid neural network to support fine cognitive abilities, including working memory, language, and symbolic thought. Interestingly, these critical periods are longer in humans than in macaque monkeys. Similarly, neuronal maturation is also longer in humans, which results in greater morphological complexity (Cupp and Uemura, 1980; Marchetto et al., 2019; Petanjek et al., 2008; Schornig et al., 2021), greater interconnectivity and finer computational processing. Together, these could contribute to the evolution of the “language-ready brain.” In the field of brain anatomy, two areas of the cerebral cortex have long been associated with language acquisition and processing – those of Broca and Wernicke. Broca’s area is involved in speech production, while speech perception is controlled by Wernicke’s area. Homologs of these two cortical areas have been found in non-human primates (Aboitiz, 2018), but both their cellular architecture and connectivity have unique characteristics in humans. Indeed, the homolog of Wernicke’s area in macaques connects to the region of the cortex close to Broca’s homolog, but not directly to it (Aboitiz and Garcia, 1997).There has been an extension of direct connections between Broca’s and Wernicke’s areas during human and ape evolution via a tract named the arcuate fasciculus. Using powerful brain imaging techniques, Rilling and colleagues have shown that the arcuate fasciculus has grown an extra connection to the temporal lobe of the human brain, potentially underpinning some of the human-specific characteristics of language (Rilling et al., 2008).
Two areas of the cerebral cortex have long been associated with language acquisition and processing – those of Broca and Wernicke. Broca’s area is involved in speech production, while speech perception is controlled by Wernicke’s area.
Development of cognitive abilities in individuals
Understanding if apes, for example chimpanzees, could ever cross the language barrier has been a fascinating question for various scientific disciplines. One way in which it has been addressed is examining the similarities between the lifetime development of an organism (ontogeny) and the evolution of the related groups of organisms (phylogeny) (Yang, 2013). In other words, it is essential to study how a subject learns to use language, but also how cultural backgrounds influence subjects. In this context, two landmark studies compared language learning in young children to language comprehension by non-human primates. Comparing the language acquisition of a bonobo named Kanzi and a female human named Alia, Savage-Rumbaugh showed that non-human primates could develop linguistic skills in a manner and timeframe comparable to those of human children (Savage-Rumbaugh et al., 1993). Furthermore, apes can develop a certain level of comprehension of human language through symbol and picture communication systems for non-verbal humans (Savage-Rumbaugh et al., 1993). However, there is no indication that non-human primates could be capable of combin- ing more than two words or symbols (Miyagawa and Clarke, 2019).
In another study involving a chimpanzee able to learn human sign language, Nim Chimpsky, Terrace concluded that the language learning and comprehension skills of chimpanzees are fairly narrow. Non-human primates can learn isolated words and their meanings; however, they have never shown an ability for the complex organization of language, nor do they combine such symbols to create new meanings (Terrace et al., 1979).
Whereas studies of ape language abilities suffer from many limitations, such as the subjective interpretation of behavioral data (Anderson, 2004), they have nevertheless allowed understanding that the language of young children is similar to the signing patterns of primates, as they both apparently result from imitation, considering the fact that they manifest limited flexibility (Yang, 2013). It is important to keep in mind that despite all these considerations, apes intentionally communicate, produce, and make use of tools to acquire food. Apes have also been reported to outperform humans in certain memory tests, possibly linked to the notion that spatial memory might be an evolutionary advantage in the wild. These studies led researchers to explore social and cultural components of language evolution.
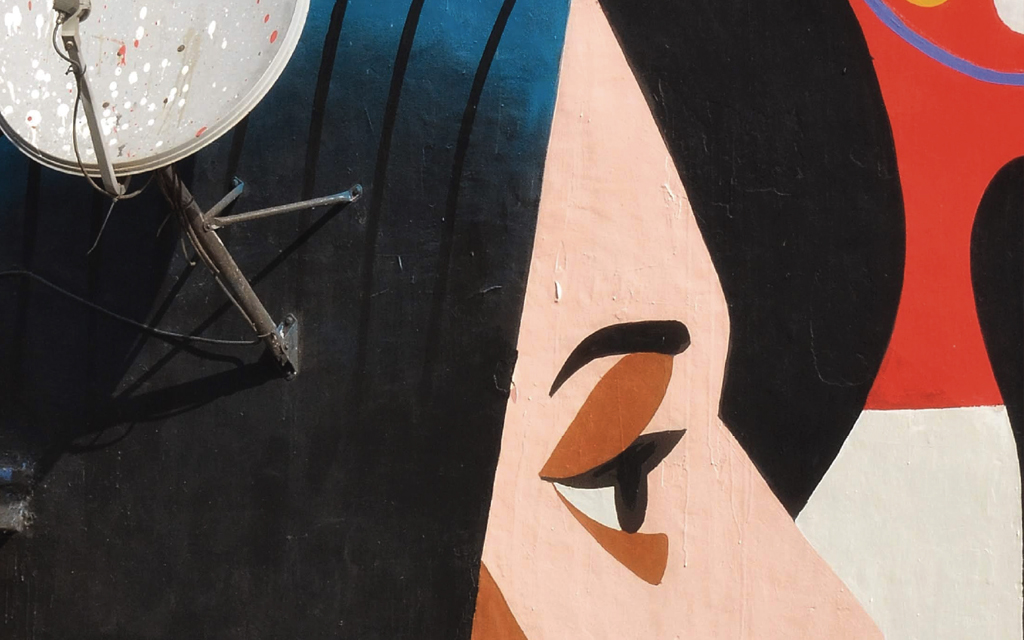
Sharing knowledge
Comparative psychology and cognitive science have highlighted several differences between the way we and other primates learn. Two features are particularly interesting: the ability to copy others and the ability to accumulate and improve knowledge over time (Tomasello, 1999). Part of our knowledge derives from copying what our peers are doing, which is obvious when we observe children. Non-human primates seem to copy less, and this could limit the speed at which knowledge spreads (Tomasello, 1999). Once knowledge is acquired, it can be improved over time, across individuals, and across generations. This phenomenon is known as cumulative cultural evolution and refers to the accumulation of sequential changes within a certain social behavior, which results in an improved function. Although culture is present in primates as well, cumulative cultural evolution is a human-specific ability that is consistently maintained across cultures. Humans do not need to start from scratch each time. Hence the advent of society allowed humans to spread knowledge faster across communities and generations. An intriguing possibility is that vocalization occurred first, and then language was instrumental in allowing humans not only to copy by looking at others but also to efficiently transfer knowledge by instructing and teaching peers.
Cumulative cultural evolution is a human-specific ability that is consistently maintained across cultures.
In conclusion, modern neuroscience enables us to study the mechanisms that shape the language-ready brain. By integrating neurobiology with societal and cultural views, we can explore the evolution of language across space and time.
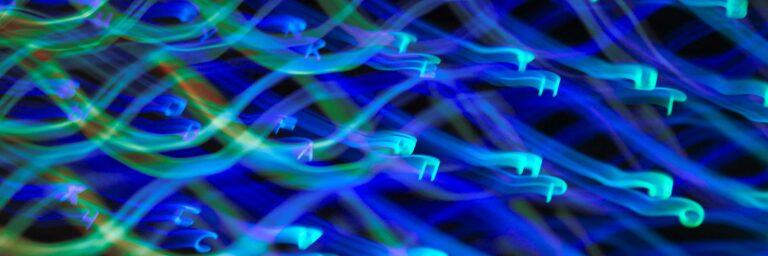
Imminent Research Grants
€100,000 to fund language technology innovators
Imminent was founded to help innovators who share the goal of making it easier for everyone living in our multilingual world to understand and be understood by all others. Each year, Imminent allocate €100,000 to fund five original research projects to explore the most advanced frontiers in the world of language services. Topics: Language economics – Linguistic data – Machine learning algorithms for translation – Human-computer interaction – The neuroscience of language.
Apply nowThe human condition seen thanks to Neanderthals
The 2022 Nobel prize for physiology and medicine was awarded to Svante Pääbo for his pioneering work on ancient DNA (Paabo, 2014). He developed a toolkit for sequencing ancient DNA recovered from small fragments of bones from caves where ancient humans lived. His work enables us to look directly at the genetic makeup of ancient humans and study what sets us apart from our closest extinct relatives. Using the tools of genetics and molecular biology, his work has brought us closer to understanding where we are from, a central question for both humanities and biological sciences. Neanderthal skulls provide us with information about the shape and size of their brains. Tools and craft artifacts found in Neanderthal caves tell us about their cognitive abilities, as crafting reflects creativity and is a proxy for what we call intelligence. Modern molecular biology and ancient DNA can shed light on the neurobiology of ancient humans, as the genetic information from ancient DNA studies can help illuminate the genetic causes of brain size and the resulting cognitive processes. To help us model the Neanderthal brain and its functions, neurobiology provides the formidable tool of brain organoids: small, three-dimensional multicellular structures that can be grown in a lab starting from human-induced stem cells and recapitulate brain development and certain features of brain function. So far, organoids have been successfully used to model human diseases and better understand how to cure them. In the context of brain evolution, organoids can also be used to model the effects of Neanderthal-specific genetic changes on brain size and function, including cellular features associated with language acquisition. Although it is unlikely that we will ever manage to study language using Neanderthal organoids directly, we may manage to learn something about the neurobiological and cellular basis of language acquisition.
Bibliography
Dennis, M. Y., Nuttle, X., Sudmant, P. H., Antonacci, F., Graves, T. A., Nefedov, M., Rosenfeld, J. A., Sajjadian, S., Malig, M., Kotkiewicz, H., et al. (2012). Evolution of human-specific neural SRGAP2 genes by incomplete segmental duplication. Cell 149, 912-922.
Enard, W., Gehre, S., Hammerschmidt, K., Holter, S. M., Blass, T., Somel, M., Bruckner, M. K., Schreiweis, C., Winter, C., Sohr, R., et al. (2009). A humanized version of Foxp2 affects cortico-basal ganglia circuits in mice. Cell 137, 961-971.
Enard, W., Przeworski, M., Fisher, S. E., Lai, C. S., Wiebe, V., Kitano, T., Monaco, A. P., and Paabo, S. (2002). Molecular evolution of FOXP2, a gene involved in speech and language. Nature 418, 869-872.
Florio, M., Albert, M., Taverna, E., Namba, T., Brandl, H., Lewitus, E., Haffner, C., Sykes, A., Wong, F. K., Peters, J., et al. (2015). Human-specific gene ARHGAP11B promotes basal progenitor amplification and neocortex expansion. Science 347, 1465-1470.
Guerrier, S., Coutinho-Budd, J., Sassa, T., Gresset, A., Jordan, N. V., Chen, K., Jin, W. L., Frost, A., and Polleux, F. (2009). The F-BAR domain of srGAP2 induces membrane protrusions required for neuronal migration and morphogenesis. Cell 138, 990-1004.
Gunz, P., Neubauer, S., Maureille, B., and Hublin, J. J. (2010). Brain development after birth differs between Neanderthals and modern humans. Current biology : CB 20, R921-922.
Hauser, M. D., Chomsky, N., and Fitch, W. T. (2002). The faculty of language: what is it, who has it, and how did it evolve? Science 298, 1569-1579.
Heide, M., Haffner, C., Murayama, A., Kurotaki, Y., Shinohara, H., Okano, H., Sasaki, E., and Huttner, W. B. (2020). Human-specific ARHGAP11B increases size and folding of primate neocortex in the fetal marmoset. Science.
Jiang, L., Wang, M., Lin, S., Jian, R., Li, X., Chan, J., Dong, G., Fang, H., Robinson, A. E., Consortium, G. T., and Snyder, M. P. (2020). A Quantitative Proteome Map of the Human Body. Cell 183, 269-283 e219.
Kalebic, N., Gilardi, C., Albert, M., Namba, T., Long, K. R., Kostic, M., Langen, B., and Huttner, W. B. (2018). Human-specific ARHGAP11B induces hallmarks of neocortical expansion in developing ferret neocortex. eLife 7: e41241.
Lai, C. S., Fisher, S. E., Hurst, J. A., Vargha-Khadem, F., and Monaco, A. P. (2001). A forkhead-domain gene is mutated in a severe speech and language disorder. Nature 413, 519-523.
MacDermot, K. D., Bonora, E., Sykes, N., Coupe, A. M., Lai, C. S., Vernes, S. C., Vargha-Khadem, F., McKenzie, F., Smith, R. L., Monaco, A. P., and Fisher, S. E. (2005). Identification of FOXP2 truncation as a novel cause of developmental speech and language deficits. Am J Hum Genet 76, 1074-1080.
Marchetto, M. C., Hrvoj-Mihic, B., Kerman, B. E., Yu, D. X., Vadodaria, K. C., Linker, S. B., Narvaiza, I., Santos, R., Denli, A. M., Mendes, A. P., et al. (2019). Species-specific maturation profiles of human, chimpanzee and bonobo neural cells. eLife 8.
Miyagawa, S., and Clarke, E. (2019). Systems Underlying Human and Old World Monkey Communication: One, Two, or Infinite. Front Psychol 10, 1911.
Neubauer, S., Gunz, P., and Hublin, J. J. (2010). Endocranial shape changes during growth in chimpanzees and humans: a morphometric analysis of unique and shared aspects. J Hum Evol 59, 555-566.
Paabo, S. (2014). Neanderthal man: in search of lost genomes. Basic books.
Petanjek, Z., Judas, M., Kostovic, I., and Uylings, H. B. (2008). Lifespan alterations of basal dendritic trees of pyramidal neurons in the human prefrontal cortex: a layer-specific pattern. Cereb Cortex 18, 915-929.
Rilling, J. K., Glasser, M. F., Preuss, T. M., Ma, X., Zhao, T., Hu, X., and Behrens, T. E. (2008). The evolution of the arcuate fasciculus revealed with comparative DTI. Nat Neurosci 11, 426-428.
Savage-Rumbaugh, E. S., Murphy, J., Sevcik, R. A., Brakke, K. E., Williams, S. L., and Rumbaugh, D. M. (1993). Language comprehension in ape and child. Monogr Soc Res Child Dev 58, 1-222.
Schornig, M., Ju, X., Fast, L., Ebert, S., Weigert, A., Kanton, S., Schaffer, T., Nadif Kasri, N., Treutlein, B., Peter, B. M., et al. (2021). Comparison of induced neurons reveals slower structural and functional maturation in humans than in apes. eLife 10.
Schreiweis, C., Bornschein, U., Burguiere, E., Kerimoglu, C., Schreiter, S., Dannemann, M., Goyal, S., Rea, E., French, C. A., Puliyadi, R., et al. (2014). Humanized Foxp2 accelerates learning by enhancing transitions from declarative to procedural performance. Proc Natl Acad Sci U S A 111, 14253-14258.
Scott, N., Neubauer, S., Hublin, J.-J., and Gunz, P. (2014). A shared pattern of postnatal endocranial development in extant hominoids. Evolutionary Biology 41, 572-594.
Sudmant, P. H., Kitzman, J. O., Antonacci, F., Alkan, C., Malig, M., Tsalenko, A., Sampas, N., Bruhn, L., Shendure, J., Genomes, P., and Eichler, E. E. (2010). Diversity of human copy number variation and multicopy genes. Science 330, 641-646.
Terrace, H. S., Petitto, L. A., Sanders, R. J., and Bever, T. G. (1979). Can an ape create a sentence? Science 206, 891-902.
Tomasello, M. (1999). The cultural origins of human cognition. Harvard University Press.
Tsui, D., Vessey, J. P., Tomita, H., Kaplan, D. R., and Miller, F. D. (2013). FoxP2 regulates neurogenesis during embryonic cortical development. J Neurosci 33, 244-258.
Vernes, S. C., Oliver, P. L., Spiteri, E., Lockstone, H. E., Puliyadi, R., Taylor, J. M., Ho, J., Mombereau, C., Brewer, A., Lowy, E., et al. (2011). Foxp2 regulates gene networks implicated in neurite outgrowth in the developing brain. PLoS Genet 7, e1002145.
Xing, L., Kubik-Zahorodna, A., Namba, T., Pinson, A., Florio, M., Prochazka, J., Sarov, M., Sedlacek, R., and Huttner, W. B. (2021). Expression of human-specific ARHGAP11B in mice leads to neocortex expansion and increased memory flexibility. The EMBO journal 40, e107093.
Yang, C. (2013). Ontogeny and phylogeny of language. Proc Natl Acad Sci U S A 110, 6324-6327.
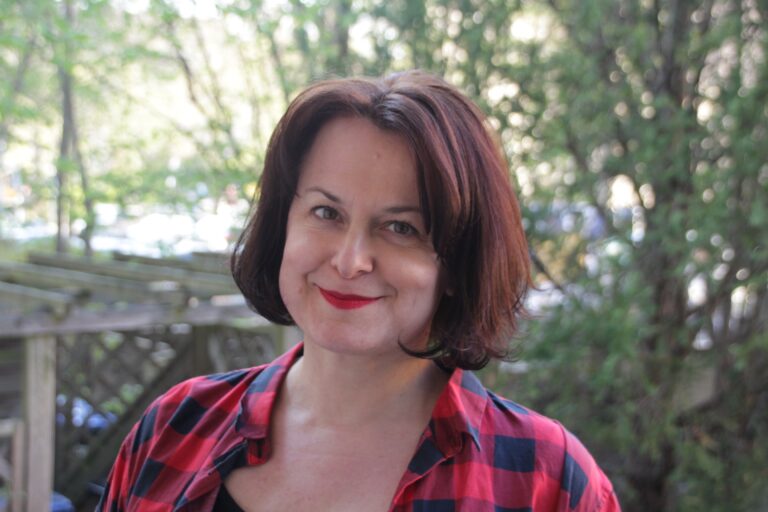
Elena Taverna
Research Group Leader at Human Technopole
Elena Taverna is a Group Leader at the Neurogenomics Research Centre. Elena obtained her degrees in Biology at the University of Milan and she completed her PhD in Pharmacology and Toxicology at the CNR’s Neuroscience Institute in Milan. From 2006 to 2015, she worked as post-doc researcher in the group of Wieland Huttner at the Max Planck Institute of Molecular Cell Biology and Genetics in Dresden, studying neural stem cells and brain development. From 2016 to March 2021 she was a Senior Staff Scientist and Project Leader at the Max Planck Institute for Evolutionary Anthropology in Leipzig, working in the team lead by Svante Pääbo. At the MPI-EVA Elena studied the evolution of neuron structure and function in apes and humans. Following up on her previous work, she also developed a robot for the high throughput analysis of single neural stem cells in tissue. At the HT, Elena uses advanced robotics combined with multimodal analysis paradigm to understand brain development and evolution at the single cell level.
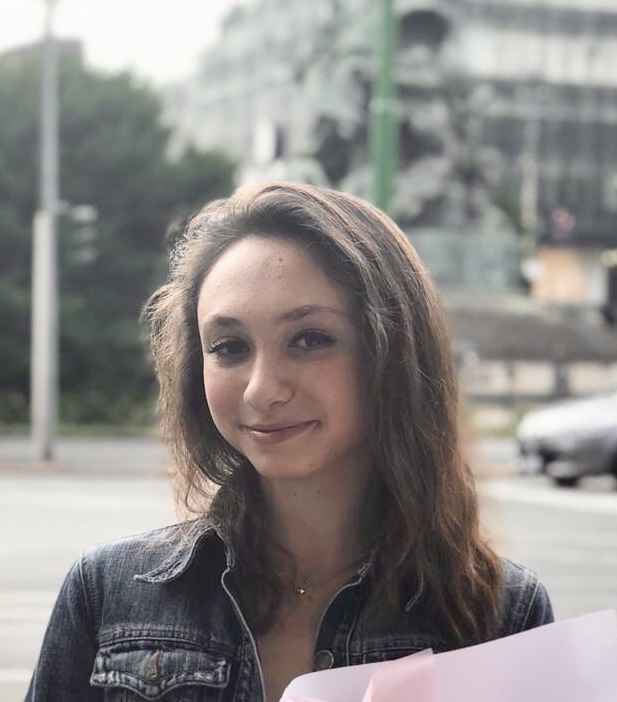
Carlotta Barelli
PhD student investigating glioblastoma stem cell biology at Human Technopole, Milan
Carlotta Barelli is a PhD student investigating glioblastoma stem cell biology at Human Technopole, Milan. Sha has graduated in neuroscience at King’s College London, where she focused on the neuroimmunology of the human fetal brain. She is also highly passionate about brain-body interactions and the use of yoga and breathwork as tools to access this connection.
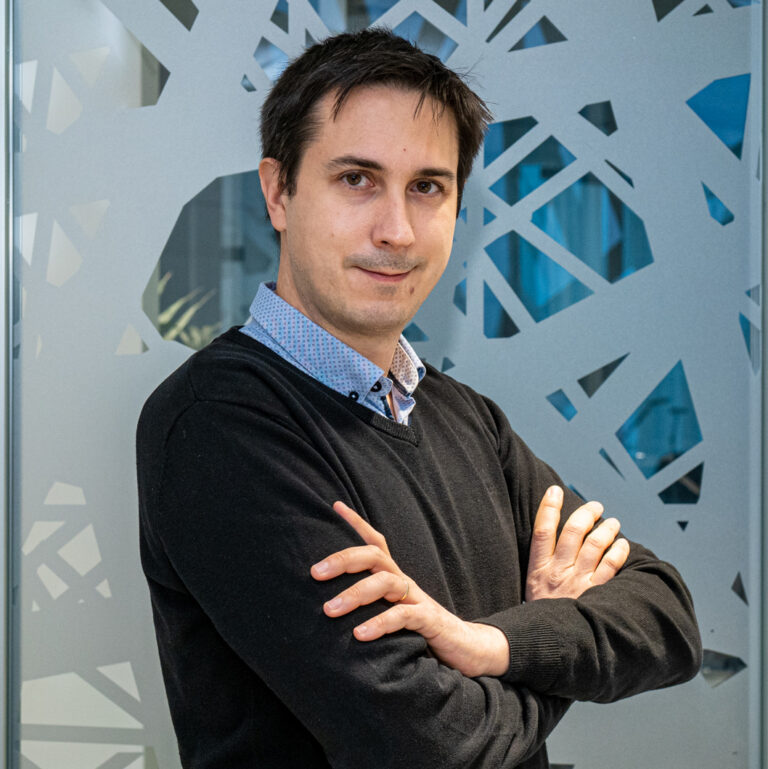
Nereo Kalebic
Research Group Leader at Human Technopole
Nereo Kalebic is a Group Leader at the Centre for Neurogenomics. He obtained his degree in Molecular Biology at the University of Zagreb in Croatia in 2007. In 2012, he completed his PhD in Molecular Biology at the European Molecular Biology Laboratory (EMBL) and Heidelberg University. During his PhD, Nereo studied the role of microtubule post-translational modifications in the development and function of the nervous system. From 2013 to 2019, he carried out post-doctoral research in Wieland Huttner’s group at the Max Planck Institute of Molecular Cell Biology and Genetics in Dresden, studying the development and evolution of the human neocortex. Nereo’s current research focuses on the molecular and cellular biological mechanisms underlying human neocortex development and their implications for human evolution and neurodevelopmental disorders.
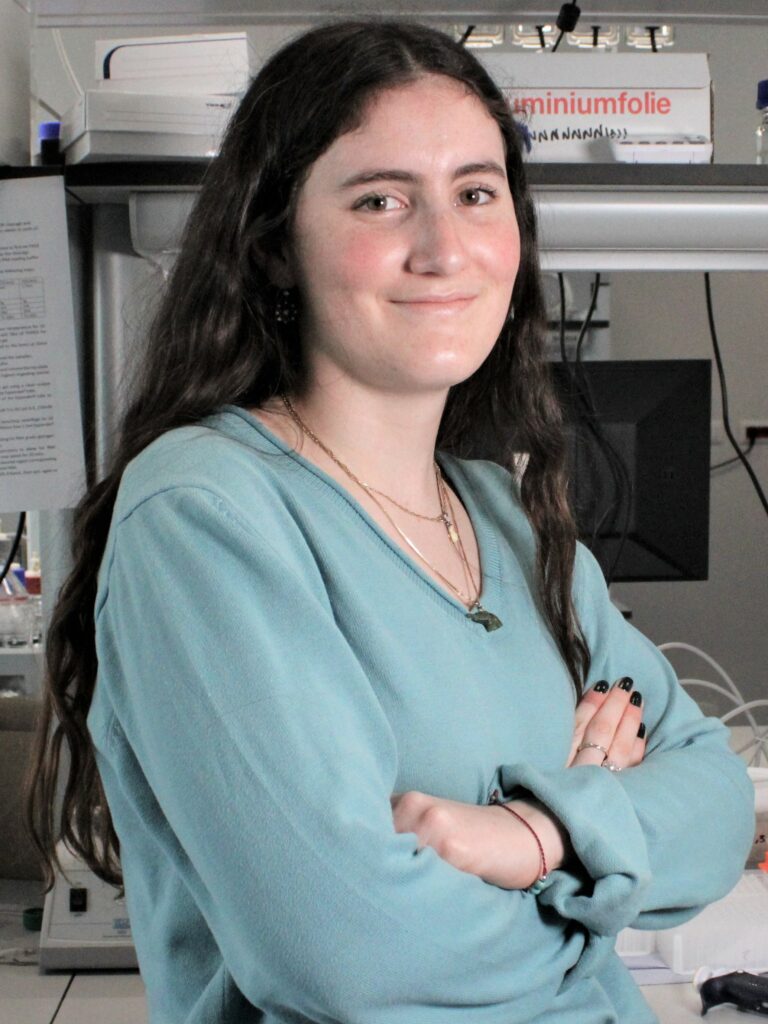
Valentina Rava
Internship at Human Technopole
Valentina Rava is an undergraduate student, she is doing her internship at Human Technopole. She has a bachelor’s degree in Medical Biotechnology, She is about to take a master’s degree in Medical Biotechnology and Molecular Medicine. At the moment, her research is mainly centered around studying neuronal maturation in non-human primates and humans.
Photo credit: Raghav Modi